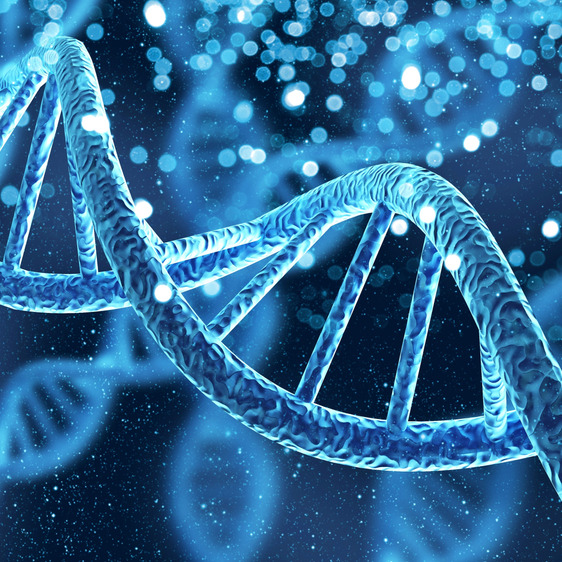
The interaction of weak electromagnetic fields with cells: A mystery of biophysics
Bioelectricity, biomagnetism and bioelectromagnetism have been shrouded in mystery and charlatanism since their discovery.
This article, based on Roland Glaser's paper "Current concepts of the interaction of weak electromagnetic fields with cells", sheds light on the scientific findings behind the interaction of weak electromagnetic fields with cells and dispels some myths.
A historical overview:
The effects of magnetism and electricity on the human body have been the subject of scientific enquiry from an early stage. While Franz Anton Mesmer's "magnetic cure" in the 18th century was still strongly characterised by mysticism, Luigi Galvani's research into "animal electricity" laid the foundations for modern electrophysiology. Despite enormous progress in physics, electrochemistry and electrophysiology, the field of bioelectromagnetism remained susceptible to charlatanism for a long time.
Scientifically founded phenomena:
Today, some phenomena in the field of bioelectromagnetism are scientifically recognised:
- Electrical excitability of cells (electrophysiology)
- Electroreception in fish and other animals
- Magnetoreception in bacteria and birds
- Passive movement of cells in artificial fields (electrophoresis, dielectrophoresis, electrorotation)
- Electrical breakthrough of membranes through short electrical impulses (cell perforation, cell fusion)
Focus on weak electromagnetic fields:
The focus today is on the effects of weak electromagnetic fields - fields with energies close to thermal noise (kT). These fields are frequently used in therapy, while at the same time there is a controversial debate about electrosmog.
How do electromagnetic fields affect cells?
The central question is: How can electromagnetic fields influence cellular processes? Numerous experiments prove that such effects exist, often occur at specific frequencies and intensities and are frequently associated with Ca²⁺ transport. Pulsed or modulated electromagnetic fields (PEMF) appear to be particularly effective when modulated at low frequencies (16-60 Hz).
The role of the cell membrane:
The cell membrane plays a crucial role in the interaction with electromagnetic fields. It acts as a diffusion barrier for ions and as a matrix for functional proteins. From an electrical point of view, it behaves like a capacitor. External fields are superimposed on the strong membrane field that exists in vivo. It is important to note that electric fields can exist in electrochemical systems without an ion current flowing.
Possible biophysical mechanisms:
there are various hypotheses on the primary mechanisms of action of electromagnetic fields on cellular structures:
- Influence on phase transitions of membrane lipid domains
- Direct influence on the function of membrane proteins (transport processes, enzymatic activities)
- Influence on the lateral organisation of the membrane and induction of lateral ion currents
- Influence on surface charges and electrical double layers
these hypotheses are based on phenomena such as cooperativity, resonance effects and the triggering of transitions between multi-stationary states.
Optimisation of the frequency bands:
the frequencies used for medical treatments are often technical and not necessarily optimal for biophysical interaction. Models predict resonance frequencies for transport proteins between 10³ and 10⁶ Hz. For therapeutic applications, a shift to the ULF, VLF, LF and MF frequency bands is therefore recommended.
Secondary biological effects:
biological effects of electromagnetic fields are caused by amplification processes. For example, the field can influence a transport protein, which leads to a change in the flow of ions and consequently to changes in cellular ion concentrations. Calcium as a secondary messenger can then trigger biochemical cascades.
Conclusion:
There is convincing evidence of the effect of electromagnetic fields on biological systems, even at energies in the range of thermal noise. However, the exact biophysical mechanisms are not yet fully understood. Further research is needed to determine the optimal frequencies and application methods for therapeutic purposes.